Galaxy formation and evolution are among the most active fields astronomical research. Small density fluctuations seeded during the epoch of inflation result in gravitational instabilities that span a wide range of physical scales. These instabilities eventually decouple from the cosmic expansion, and collapse to form gravitationally-bound structures that possess a wide variety of physical attributes: starburst galaxies, giant ellipticals, grand design spirals and black hole-fueled active galactic nuclei are just a few examples of the rich variety of emergent structure.
Galaxy formation and evolution are highly non-linear and involve a complex interplay of a variety of physical processes that affect not only what happens within galaxies, but also to matter at very large distances, well beyond their boundaries. This is because of physical processes — such as energetic feedback from stars and supermassive black holes — that are so powerful that they shape the fate of matter millions of lightyears from their source. Galaxy formation also involves a wide range of physical scales: gravitational tides from surrounding structures torque mass that accretes onto galaxies giving rise or ordered rotation, while stars — the cellular building blocks of galaxies — form on scales a billion times smaller.
These processes are best captured by cosmological hydrodynamical simulations, which attempt to self-consistently model each of the relevant physical processes, from the linear collapse of primordial instabilities to the wealth of emergent structures. Such simulations now achieve an unprecedented level of sophistication and realism that allows us to study the internal properties of galaxies, how they emerge and evolve, and their connection to the large scale structure of the Universe. Researchers at ICRAR/UWA are helping design the next generation of hydrodynamical simulations together with an international team of researchers (including scientists from Australia, The Netherlands, United Kingdom, Canada, Finland and other European countries). These projects include “EAGLE-XL”, which builds on the extremely successful EAGLE project, and the Genesis simulation suite (led by Professor Chris Power), among several others.
The analysis and interpretation of these simulations offer a number of potential PhD projects that can also be tailored to the interests of the student:
- The violent and chaotic early Universe: understanding how the early epoch of active star formation and interactions shape the properties of galaxies as we observe them today.
- Hydrodynamical effects in galaxy groups and clusters throughout cosmic time and how they affect galaxy properties.
- The effect of baryon physics on the large scale structure of the Universe.
- The formation of galaxy disks and bulges over cosmic time.
- The multiscale nature of angular momentum: from the cosmic web to galaxies.
- The baryon cycle: interplay between gas accretion and stellar and black-hole driven feedback.
- The metal Universe: building up of the metal content of the intergalactic medium and galaxies.
- The low surface brightness Universe and its connection to galaxy assembly and baryon physical processes.
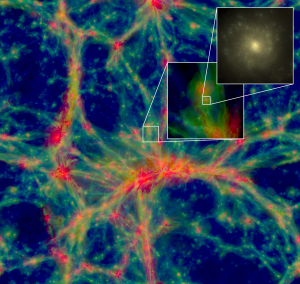
The image above is a slice through the simulation volume, with the intergalactic gas colour coded from blue to green to red with increasing temperature. Hot gas has temperatures of more than 100,000K, and is contained with dark matter structures that host galaxies. Such hot gas can be detected in X-rays. The insets zoom into a galaxy like the Milky Way, showing first its gas, and then its beautiful stellar disc: it looks remarkably similar to observed spirals. Credit: EAGLE team.
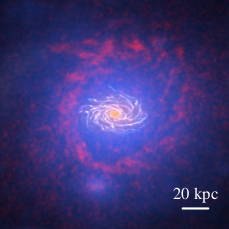
Example galaxy in the NIHAO simulations showing the distribution of the gas and stars. Credit: D. Obreschkow.