ICRAR’s science program is based on the instinctive human endeavour to understand more about the nature of everything around us.
Making sense of the information from new generation telescopes such as the Australian Square Kilometre Array Pathfinder (ASKAP), the Murchison Widefield Array (MWA) and the Square Kilometre Array (SKA) will vastly expand our view and understanding of the Universe. By attracting a diverse team of world-class researchers and collaborating with other leading institutions, ICRAR has grown into an internationally-renowned centre of astronomy research since its inception in 2009. We have published over 1800 peer-reviewed journal articles.
ICRAR’s Peer-reviewed Publications
Science Focus
Our science programme is divided across ICRAR’s two nodes, Curtin and UWA, although some of our projects involve a high level of collaboration between the two nodes. Curtin’s key focuses are extragalactic radio science, accretion physics and slow transients, the epoch of reionisation, and pulsars/fast transients. Our UWA node is studying galaxies in the local and distant Universe, and cosmological theory. The overall program combines large observational programmes with large theoretical simulations to explore the Universe and pave the way for exciting SKA science.
Extragalactic Radio Science
Project lead: Dr Natasha Hurley-Walker
This project exploits the superb multi-frequency survey capabilities of the Murchison Widefield Array in order to conduct novel extra-galactic science with particular focus on:
- Characterising radio clusters and the cosmic web;
- Unravelling the astrophysics of the life-cycles of radio galaxies and tracing their evolution across cosmic time; and
- Using the phenomenon of inter-planetary scintillation over large areas to study distant radio galaxies and learn about the solar wind.
Case study: GLEAM
Accretion Physics and Slow Transients
Project lead: Prof James Miller-Jones
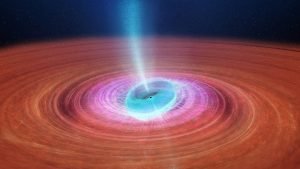
Artist’s impression of the inner parts of the accretion disk in V404 Cygni. The black hole rotates about a different axis to the binary orbit. As the spinning black hole drags spacetime around with it, the puffed-up inner accretion disk wobbles around like a spinning top. The jets launched from the innermost parts of the flow are redirected, either by the puffed-up inner disk or the strong winds being driven off it by the intense radiation. Credit: ICRAR
Short-lived explosive events such as the deaths of massive stars, or the outbursts caused by a black hole feeding on a normal star, provide excellent laboratories for studying high-energy astrophysics, allowing us to probe energies, magnetic fields, and strong gravity that can never be reproduced here on Earth. This project uses radio telescopes (including the precursor facilities to the Square Kilometre Array) to characterise the jets and outflows produced in these extreme environments, determining how they are launched and how they feed back energy to their surroundings. This work benefits from the advent of multi-messenger astronomy, using gravitational waves, neutrinos, and high-energy gamma-rays to provide new windows into these cosmic cataclysms. Finally, the project uses the techniques developed for the study of transient astrophysical events to develop the Murchison Widefield Array’s capabilities for detecting and tracking objects in low-Earth orbit.
Fast Transients and Pulsars
Project lead: Dr Ramesh Bhat
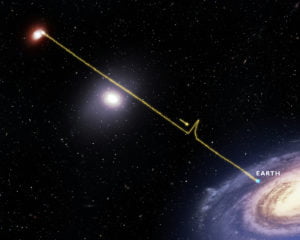
An artist’s impression of Fast Radio Burst 181112 travelling through the halo of a galaxy 4 billion light-years from Earth. Credit: © J. Josephides, Centre for Astrophysics and Supercomputing, Swinburne University of Technology.
The impulsive emission from pulsars and short-timescale transients, such as fast radio bursts, is associated with the most energetic and brightest single events in the Universe. This emission represents Nature’s ultimate laboratory, since it is usually generated by matter under extreme conditions whose properties probe physical regimes that far transcend the range achievable in terrestrial experiments. Our objectives are to both understand the physics behind these phenomena and to use them as lighthouses to probe the Universe through which their ultra-luminous emission propagates. We are using fast radio bursts to weigh the Universe and to probe the very properties of space-time over a large range of the Universe’s history.
We are using fast radio bursts to weigh the Universe to probe the properties of space-time over a large range of the Universe’s history, and pulsars as astrophysical tools for testing strong-field gravity and as detectors of gravitational waves.
The Local Universe
Project lead: Dr Barbara Catinella
This project will study the origin and evolution of galaxies by taking advantage of the exquisitely detailed observations that can be obtained for objects in the local Universe. Surveys carried out with cutting-edge radio telescopes (ASKAP, MWA, ALMA), aided by state-of-the-art observations at other wavelengths (e.g., 8m-class ESO telescopes), will shed light on the various components of the interstellar medium of nearby galaxies, and its connection with stellar and star-formation properties, as well as surrounding environment. In particular, WALLABY is designed to detect large numbers of previously unknown galaxies in the local Universe, and to study the interaction of the outskirts of galaxy disks with the intergalactic medium for more distant galaxies, thus improving understanding of accretion, interaction and outflows in galaxies in general.
THE DISTANT UNIVERSE
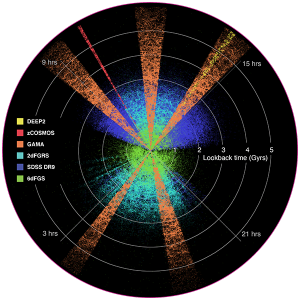
Each coloured dot on this diagram is a galaxy that has been observed as part of one of many survey projects conducted globally. Credit: Simon Driver and the GAMA team.
Project lead: A/Prof Aaron Robotham
The Distant Universe group is focussed on understanding how mass in the Universe has transformed from a smooth distribution of ionised atoms 13 billion years ago, to the myriad of galaxy types distributed in clusters and filamentary chains that we can see today. Underpinning this is the story of the growth of dark matter and the emergence of dark energy, which work together to drive the formation and evolution of galaxies and all that lies within. In more Physics terminology we’re interested in the growth of mass (gas, stars, dust and black-holes), the production of energy (star-formation and accretion), and the redistribution of angular momenta (rotation), as baryons collapse inside dark matter halos. These are the locations where hot gas cools, molecular gas forms, stars are created, which then produce dust and some of which explode producing supermassive black holes. Most of our work is observational and we use some of the world’s largest ground and space-based facilities to measure the radiation from galaxies across the full electro-magnetic spectrum, as well as measuring distances and spatially resolved motions, i.e., rotations and dispersions.
Central to the group’s work are our redshift measurements and we lead a number of large spectroscopic campaigns that we use to turn our two-dimensional images into three dimensional maps of the Universe. In particular ICRAR and UWA have invested heavily in the European Southern Observatory’s 4-metre Multi-Object Spectroscopic Telescope from which we will measure the distances to over 2 million galaxies from 2023 onwards. The group is also closely involved in a number of space-telescopes including: the Hubble Space Telescope, eROSITA, the James Webb Space Telescope, Euclid space telescope and the WFIRST space telescope all of which will be providing cutting-edge data over the next few years.
Cosmological Theory
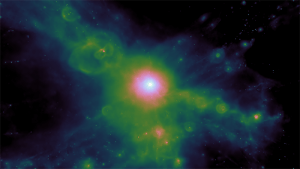
This simulation shows an energetic galaxy and the powerful X-rays emitted by the gas within it. Bubbles in the gas caused by supernovae and stellar winds are also visible. Credit: Chris Power, Rick Newton and the ICRAR Simulations Team.
Project lead: A/Prof Chris Power
The Cosmological Theory group combines large-scale supercomputer simulations and state-of-the-art theoretical models to tackle fundamental questions in galaxy formation and cosmology, the predictions from which are used to create mock observables for current and future galaxy surveys. Key science questions focus on how galaxies assemble their mass and angular momentum; how feedback from stars and black holes shape their host galaxies; how the underlying cosmological model impacts galaxy formation; and how theories of dark matter can be tested observationally in a robust fashion. Close synergies with observational projects allow researchers in Cosmological Theory to support the astrophysical interpretation of galaxy surveys, such as ASKAP WALLABY and DINGO; SAMI; DEVILS; and WAVES on 4MOST, whilst also allowing for the refinement and extension of theoretical models. Our researchers exploit a range of techniques, including numerical and hydrodynamical simulations; semi-analytic modelling; semi-empirical modelling, and have developed an extensive suite of novel software, including VELOCIraptor (Elahi et al. 2019), a 6D phase space structure finding algorithm; the Shark semi-analytical model of galaxy formation (Lagos et al. 2018); and SimSpin (Harborne et al. 2019), a mock IFU datacube generator. Cosmological Theory also collaborates closely with national and international teams, including the Australian ARC Centre of Excellence for All-Sky Astrophysics in 3D (ASTRO 3D) and the European EAGLE/EAGLE-XL Simulation team.
Epoch of Reionisation
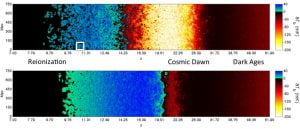
Two different possible evolutionary paths of neutral gas in the Universe. These paths are being probed by low-frequency radio telescopes, such as the Murchison Widefield Array. Image courtesy of Andrei Mesinger.
Project lead: A/Prof Cathryn Trott
The Epoch of Reionisation project explores the first billion years of the Universe, as probed through the redshifted emission line of neutral hydrogen gas. Studying the spatial and temperature distribution of the neutral hydrogen gas between the first galaxies provides key insights into the growth of structure at the Cosmic Dawn, and the first sources of ionising radiation in the Universe. We are exploring the spatial nature of this signal with the Murchison Widefield Array (MWA), and future Square Kilometre Array (SKA), and also pursuing the globally-averaged signal with short-spacing interferometers. Beyond the observational data, we also explore the astrophysical interpretation of the signal, and develop and apply new tools to observing this era of the Universe.