From a black hole to the South Pole: Scientists identify first neutrino from a tidal disruption event
Tracing back a ghostly particle to a shredded star, scientists have uncovered a gigantic cosmic particle accelerator. The subatomic particle, called a neutrino, was hurled towards Earth after the doomed star came too close to the supermassive black hole at the centre of its home galaxy and was ripped apart by the black hole’s colossal gravity. It is the first particle that can be traced back to such a ‘tidal disruption event’ (TDE) and provides evidence that these little understood cosmic catastrophes can be powerful natural particle accelerators, as the team including ICRAR-Curtin’s Professor James Miller-Jones reports in the journal Nature Astronomy. The observations also demonstrate the power of exploring the cosmos via a combination of different ‘messengers’ such as photons (the particles of light) and neutrinos, also known as multi-messenger astronomy.
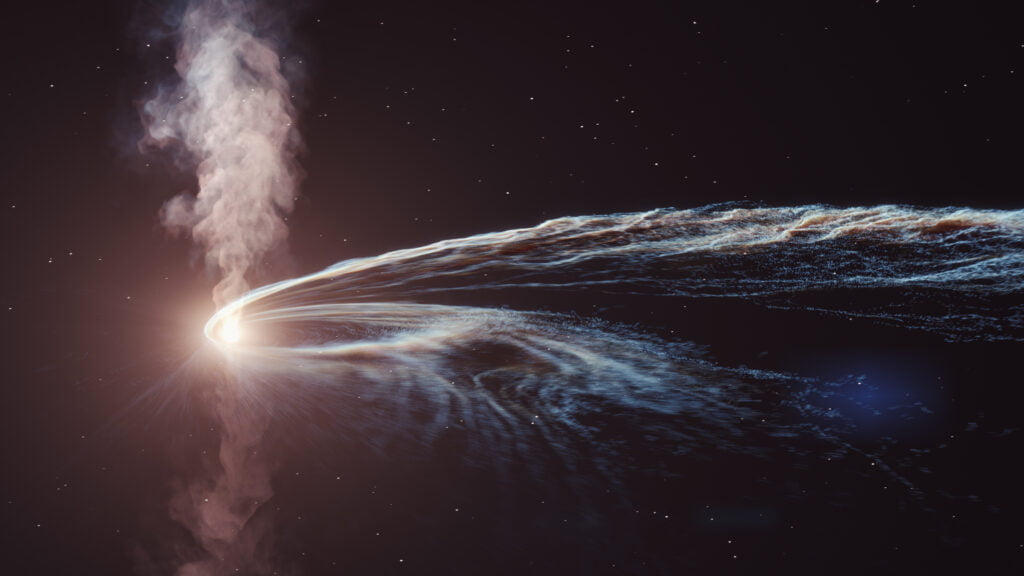
Smoking gun: After the supermassive black hole tore the star apart, roughly half of the star debris was flung back out into space, while the remainder formed a glowing accretion disc around the black hole. The system shone brightly across many wavelengths and is thought to have produced energetic, jet-like outflows perpendicular to the accretion disc. A central, powerful engine near the accretion disc spewed out these fast subatomic particles. Credit: DESY, Science Communication Lab
“The combined observations demonstrate the power of multi-messenger astronomy,” says co-author Marek Kowalski, head of neutrino astronomy at DESY and a professor at Humboldt University in Berlin. “Without the detection of the tidal disruption event, the neutrino would be just one of many. And without the neutrino, the observation of the tidal disruption event would be just one of many. Only through the combination could we find the accelerator and learn something new about the processes inside.”
The neutrino began its journey around the time the first animals developed on Earth, travelling 700 million years from the far-away, unnamed galaxy in the constellation Delphinus (the Dolphin) to finally arrive at the IceCube neutrino detector at the South Pole. Scientists estimate that the enormous black hole responsible for launching this neutrino is as massive as 30 million suns. “The force of gravity gets stronger and stronger, the closer you get to something. That means the black hole’s gravity pulls the star’s near side more strongly than the star’s far side, leading to a stretching effect,” explains lead author Robert Stein, DESY scientist. “This difference is called a tidal force, and as the star gets closer, this stretching becomes more extreme. Eventually it rips the star apart, and then we call it a tidal disruption event. It’s the same process that leads to ocean tides on Earth, but luckily for us the moon doesn’t pull hard enough to shred the Earth.”
About half of the star’s debris was flung into space, while the other half settled on a swirling disc around the black hole. This ‘accretion disc’ is somewhat similar to the vortex of water above the drain of a bathtub. Before plunging into oblivion, the matter from the accretion disc gets hotter and shines brightly. This glow was first detected by the Zwicky Transient Facility (ZTF) on Mount Palomar in California on 9 April 2019, and the outflow lasted hundreds of days.
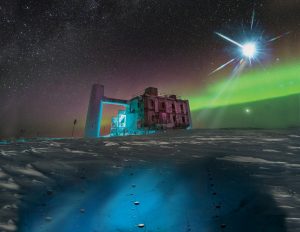
In this artistic rendering, based on a real image of the IceCube Lab at the South Pole, a distant source emits neutrinos that are detected below the ice by IceCube sensors, called DOMs. Credit: IceCube/NSF
Six months later, on 1 October 2019 the IceCube neutrino detector at the South Pole registered an extremely energetic neutrino from the direction of the tidal disruption event. The extremely lightweight neutrinos hardly interact with anything, able to pass unnoticed through not just walls but whole planets or stars, and are hence often referred to as ghost particles. So, even catching just one high-energy neutrino is already a remarkable observation. The detection prompted further observations of the event with many instruments across the electromagnetic spectrum, from radio waves to X-rays.
ICRAR-Curtin’s Professor James Miller-Jones worked on the design and analysis of the radio observations with the Karl G. Jansky Very Large Array that provided the key evidence for, and determined the physical parameters of, the outflow. Researchers think it was the long-lasting outflow that launched the neutrino.
“Not all tidal disruption events seem to produce such energetic outflows,” says Professor Miller-Jones. “The radio detection of this event therefore provided important evidence that it was likely the source of the neutrino.”
Lead author Robert Stein explains that “This is the first neutrino linked to a tidal disruption event, and it brings us valuable evidence. Tidal disruption events are not well understood. The detection of the neutrino points to the existence of a central, powerful engine near the accretion disc, spewing out fast particles. And the combined analysis of data from radio, optical and ultraviolet telescopes gives us additional evidence that the TDE acts as a gigantic particle accelerator.”
“We might only be seeing the tip of the iceberg here. In the future, we expect to find many more associations between high-energy neutrinos and their sources,” says Francis Halzen, Professor at the University of Wisconsin-Madison and Principal Investigator of IceCube, who was not directly involved in the study. “There is a new generation of telescopes being built that will provide greater sensitivity to TDEs and other prospective neutrino sources. Even more essential is the planned extension of the IceCube neutrino detector, that would increase the number of cosmic neutrino detections at least tenfold.” This TDE marks only the second time, a high-energy cosmic neutrino could be traced back to its source. In 2018, a multi-messenger campaign presented an active galaxy, the blazar TXS 0506+056, as the first ever identified source of a high-energy neutrino, recorded by IceCube in 2017.
“It’s really exciting for the radio astronomy expertise of Australian-based astronomers to be instrumental in uncovering these new insights into the high-energy Universe. The huge leap in sensitivity that will be provided by the Square Kilometre Array will allow detailed studies of more of these cataclysmic events. This will provide critical new insights into the workings of black holes.” Professor Miller-Jones said.
As the star approaches the black hole, the enormous tidal forces stretch it more and more until it is finally shred. Half of the stellar debris is flung back into space, while the remaining part forms a rotating accretion disk from which two strong outflows of matter shoot up and down. The system acts as a powerful natural particle accelerator. Scientists have caught a single neutrino hurled towards Earth by the system. Animation: DESY, Science Communication Lab
The IceCube Neutrino Observatory is a facility of the U.S. National Science Foundation operated at the Amundsen-Scott South Pole Station under the U.S. Antarctic Program. Apart from IceCube and ZTF, the instruments Spectral Energy Distribution Machine (SEDM), Palomar 200-inch Hale Telescope (P200), Liverpool Telescope (LT), NASA’s Neil Gehrels Swift Observatory, Lowell Discovery Telescope, Lick Observatory Shane Telescope, Keck Telescope, ESA’s X-ray Multi-Mirror Mission (XMM-Newton), Karl G. Jansky Very Large Array (VLA), AMI Large Array (AMI-LA), MeerKAT, and NASA’s Fermi Large Area Telescope (Fermi-LAT) provided observational data for the study.
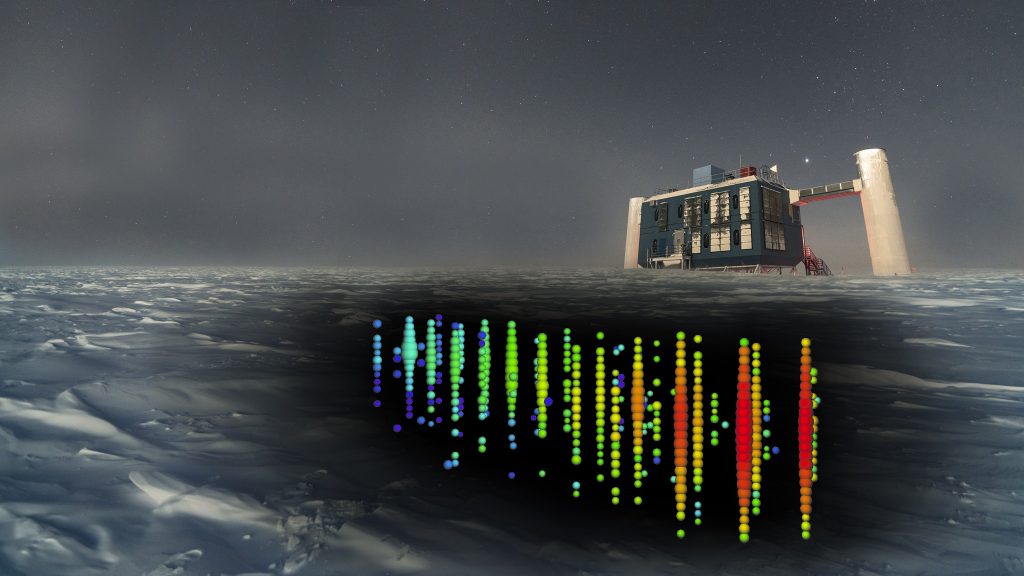
The IceCube Neutrino Observatory is buried at depths between 1.5 and 2.5 kilometers below the South Pole. The only visible equipment is the IceCube Lab, also called the ICL, which hosts the computers that collect data from the over 5,000 light sensors in the ice. In this artistic rendering, based on a real image of the ICL, neutrino event IC170922 is shown in the ice below Antarctica’s surface. Credit: IceCube Collaboration/NSF
Original publication:
A tidal disruption event coincident with a high-energy neutrino; Robert Stein, Sjoert van Velzen, Marek Kowalski, et al.; Nature Astronomy, 2021, DOI: 10.1038/s41550-020-01295-8